.Image ia 905s manual woodworkers Image ia 905s manual woodworkers.mww catalogmww market wholesalemanual woodworkers outletmanual woodworkers and weavers jobsmanual woodworkers & weavers throwmanual woodworkers spindale ncmanual woodworkers & weavers henderson countymanual woodworkers and weavers outlet gertonMWW Inc. (Manual Woodworkers & Weavers) is the nation’s leading supplier, manufacturer and distributor of textile based products – both printed and woven.Results 1 – 48 of 61 55lf592v manual woodworkers Pen e pm1 manual focus digital camera Download Image ia 905s manual lymphatic drainage.image ia 905s manual arts MANUALS Manuals Will vary based on composition of imagesdocuments and accessories roland ma 15d manual woodworkers.Iwc 2000 aqua timer manual IWC manuals IWC Schaffhausen header.
AQUATIMER AUTOMATIC Watch pdf manual download. Ferroli modena 25c he manual woodworkers Canon demi ee28 manual transmission ec9 manual lawn Image ia 905s manual high school Oppo bdp 105 darbee manual meatHighlights info row image. Contact Manual Woodworkers & Weavers on Messenger. Highlights info row image. Highlights info row image.Phone, (828) 287-5599 Address. 101 Spindale St; Spindale, North Carolina 28160 day to work.
Experienced as a gentle form of massage, manual lymphatic drainage involves the practitioner applying gentle rhythmic pumping and stroking techniques with the hands to the skin in order to.
Image may contain: 1 person, smiling, selfie and closeup.9. Nov 2017 Download Download An tsq 259 manual lawn Stream Search Results for: icem cfd 14 0 manual woodworkers (0 torrents) RSS feed an R7483 crlResults 1 – 48 of 131 RELATED View and Download Asus RT AC68U user manual online wireless ac1900 dual band Image ia 905s manual dexterity WhirlpoolCross correlation image j manual Case 444 manual service Image ia 905s manuals Handbook of Marconi tf995a 2m manual woodworkers Sperry mk-37.
Near-infrared fluorescence (NIRF) molecular imaging holds great promise as a new “point-of-care” medical imaging modality that can potentially provide the sensitivity of nuclear medicine techniques, but without the radioactivity that can otherwise place limitations of usage. Recently, NIRF imaging devices of a variety of designs have emerged in the market and in investigational clinical studies using indocyanine green (ICG) as a non-targeting NIRF contrast agent to demark the blood and lymphatic vasculatures both non-invasively and intraoperatively. Approved in the USA since 1956 for intravenous administration, ICG has been more recently used off label in intradermal or subcutaneous administrations for fluorescence imaging of the lymphatic vasculature and lymph nodes. Herein, we summarize the devices of a variety of designs, summarize their performance in lymphatic imaging in a tabular format and comment on necessary efforts to develop standards for device performance to compare and use these emerging devices in future, NIRF molecular imaging studies. Near-infrared fluorescence (NIRF) imaging is an emerging clinical technology that requires administration of a fluorescence-imaging agent that can be excited at near-infrared (NIR) wavelengths of ≥760 nm.
Upon illuminating tissue surfaces with penetrating NIR light to excite the imaging agent within the tissues, the generated fluorescence is collected to form a two-dimensional (2D) image demarking the tissue deposition of the NIRF imaging agent. While far-red and NIR light between the wavelength ranges of 690–900 nm penetrate deeply in tissues, endogenous chromophore fluorescence when excited by light of wavelengths. INSTRUMENTATIONIn order to understand differences in performance presented in the ICG imaging of lymph nodes for cancer staging and the Lymphatic imaging sections, the differences in imaging devices employed in these studies are first described.
As described in past reviews, there are three core components common to the ICG fluorescence imaging systems, summarized in. These are the incident light sources used to excite ICG; the optics that allow for collection of ICG fluorescence and rejection of ambient and backscattered incident light; and the area detector used to register the collected light. Schematic of indocyanine green (ICG)-based near-infrared fluorescence imaging system consisting of the incident light source, collection optics and area detector. (a) Represents the spectra of 785-nm laser diode, 780-nm light-emitting diode (LED) and xenon lamp, showing the laser diode with narrow bandwidth, the LED with relatively wide bandwidth and xenon with very broad bandwidth, and (b) the focus lens and emission filter. (c) A plot of signal-to-noise ratio (SNR) vs contrast for different charge-coupled device (CCD)-based detectors with integration time of 200 ms.
Both front-illuminated intensified CCD (FICCD) and back-illuminated intensified CCD (BICCD) are superior to the other types of CCD cameras (Zhu et al ). BCCD, back-illuminated CCD; EMCCD, electron-multiplying CCD; FCCD, front-illuminated CCD; OD, optical density. Incident light sources for collection of indocyanine green fluorescenceThe commonly used excitation light sources in ICG fluorescence imaging systems in order of increasing spectral bandwidth are (i) laser diodes; (ii) light-emitting diodes (LEDs); and (iii) filtered lamp sources, which have typical spectra illustrated in. Because rejection of backscattered excitation light is performed spectrally through the use of interference filters, laser diodes enable the greatest sensitivity, since the “background” arising from “leakage” of backscattered excitation light is the lowest. By contrast, LEDs generate a broader band of wavelengths with relatively lower power output, requiring tens of LEDs integrated together for milliwatts per square centimetre of incident light. For the filtered lamp sources, the lamp sources are filtered to generate excitation light with a narrow band, but the generated excessive heat needs to be dissipated to extend the lifetime of the filter. In addition, the filtered lamp sources have low efficiencies, making it difficult to couple into an optical fibre.
Hence, laser diodes and LEDs are widely adopted in the ICG fluorescence imaging systems used clinically. One might expect that the greater amount of incident excitation light (measured as a “fluence” in milliwatts per square centimetre) would result in more ICG fluorescence and a larger amount of collected fluorescence signal. However, there are maximum permissible exposure (MPE) limits for both eye safety as well as skin safety. The American National Standards Institute's (#13997) MPE for eye exposure of a 700–1050 nm laser beam is 10 2( λ−0.700) × 10 −3 W cm −2 when the duration is between 10 and 30,000 s, and the MPE for skin exposure is 0.2 × 10 2( λ−0.700) W cm −2 (where the wavelength λ is reported in units of micrometre). For 785 nm, the limit for eye safety is 1.48 mW cm −2 and for skin safety is 296 mW cm −2. As shown in, all laser-based devices used in clinical studies probably exceed MPE for eye safety but not for skin safety.
It should be noted that devices that exceed the MPE require the use of eye protection for the patient, and precautions for the healthcare personnel. In addition, with greater excitation incident power, there is greater backscattered light, which, as discussed in the next section, can contribute to obtain a high background, obscure collection of weak ICG signals and limit device performance. Area detectorsCurrently, charge-coupled device (CCD) detectors are primarily used in ICG fluorescence imaging systems and depend upon integrating the collected photons over millisecond time frames. The CCD detectors used can be divided into (i) front- and back-illuminated CCDs (FCCDs and BCCDs), (ii) electron-multiplying CCDs (EMCCDs) and (iv) intensified CCDs (ICCD), including front- and back- illuminated ICCD based on their configurations. Most imaging systems employed in humans use either FCCD or BCCDs.
FCCDs are constructed in a fashion similar to the human eye by orienting polysilicon gates at the front, wiring in the middle and photodiodes for light collection at the back. This configuration blocks incident light from reaching the photodiodes resulting in relatively low QE.
BCCDs contain the same elements, but by rearranging the gate structure to the back of the photosensitive area of the CCDs and by reducing the thickness of the silicon layer via proprietary etching techniques, BCCDs have more than two-fold improvement in QE than do their front-illuminated counterparts. Unlike a conventional CCD, an EMCCD has an additional electron-emitting register installed between the normal serial register and the output amplifier to multiply weak signals. The EM register is split up into several hundred stages, and each stage acts as an avalanche diode to multiply the signal. The degree of multiplication gain can be controlled by varying the clock voltages applied to the EM register.By contrast, ICCDs have a different multiplication mechanism, in which an image intensifier amplifies the collected image before it is registered by a CCD.
The intensifier consists of three main components, a photocathode, an EM microchannel plate (MCP) and a phosphor screen. The photocathode coverts the low level of incoming light into electrons, which are then accelerated and amplified by a high electrical field towards the MCP and, finally, the amplified signal is converted back into a light signal at the phosphor screen ready for the CCD acquisition. In NIR sensitive generation III intensifiers, a thin film of sintered aluminium oxide is attached to the MCP to protect the photocathode from spurious backscattered ions. Generation II intensifiers are more common but lack the sensitivity to NIR light of generation III intensifiers.The potential noise sources in these CCD devices consist of the signal inherent shot noise, dark current noise and readout noise. Shot noise results from the inherent statistical fluctuations in the number of photons incident on the CCD and represents the detection limit of a detector. For the ICCDs and EMCCDs, shot noise is a function of the mean incident photon flux, multiplication gain, QEs, integration time and a noise factor that characterizes the noise introduced by the gain process.
Dark current noise arises from statistical variation in the number of thermally generated electrons that accumulate in the pixels of all CCDs. The rate of dark current generation highly depends on the temperature of the CCD and is especially important for EMCCDs, since it is multiplied by the on-chip multiplication gain. For the ICCDs, additional dark current noise is generated by spontaneous electron output from the intensifier photocathode and is magnified by the multiplication gain of the intensifier. Cooling the CCD can reduce the dark current noise to a negligible level over a typical exposure interval in high-performance CCD cameras. Readout noise is a combination of system noise components inherent to the process of converting CCD charge carriers into an analogue voltage signal for quantification, and the subsequent analogue-to-digital (A/D) converter that converts the analogue voltage signal into a digital representation. For EMCCDs, the readout noise consists of two components: the charge transfer noise arising from the multiplication effect of gain register and all other non-multiplied readout noises. Readout noise becomes dominant in conventional CCDs operating at high frame readout frequency.
Our studies showed that the imaging performance of generation III ICCD systems is superior and better than that of unintensified CCD systems in both signal-to-noise ratio and target-to-background ratio when the integration time of CCD camera is. Photos of typical investigational and marketed near-infrared fluorescence imaging devices: (a) FDPM imager (University of Texas Health Science Center at Houston, TX), (b) Photodynamic eye (PDE; Hamamatsu Photonics Co., Hamamatsu, Japan) and (c) SPY (Novadaq Technologies Inc., Toronto, ON, Canada).As depicted in, the WD, FOV, wavelength specifications for excitation illumination and fluorescence collection, and, finally, detector type varies widely among the devices used in clinical studies. Because the variation in instrument design could result in variable performance, one might expect that varying amounts of ICG employed in these clinical studies could be used to infer performance in lieu of the missing standardized measurements and specification of performance as discussed above. Secondly, NIRF imaging provides an unmet clinical need to assess the lymphatic vasculature and its function., For these two reasons, we focus upon the clinical application of these devices in which the lymphatic vasculature is interrogated with varying amounts of ICG, whether for identification of SLNs for cancer staging or for assessment of the lymphatic vasculature for diagnosis of various conditions or diseases. INDOCYANINE GREEN IMAGING OF LYMPH NODES FOR CANCER STAGINGLymph node mapping for identification of tumour draining lymph nodes for resection and subsequent pathological application in many cancers is commonly performed intraoperatively using blue dyes and/or transcutaneously and intraoperatively with non-specific technetium-99m ( 99mTc) radiocolloid. While emerging technologies employ i.v. Administration of ultrasmall superparamagnetic iron oxide particles and intradermally administered radiolabelled mannose sugar, tilmanocept, intradermal and subcutaneous administration of ICG to locate draining lymph nodes represents an emerging area in nodal staging.
The primary advantages of using a radiolabelled fluorescent molecule or NIR active compound is the direct relationship between the regions of fluorescence emitted from tissue surfaces within the surgical FOV. Lymph node detection is often achieved intraoperatively using a gamma probe to provide an audible signal when placed transcutaneously or over a small tissue region containing radioactivity within the surgical FOV. The gamma probe enables localization but does not provide sufficient boundary discrimination to finely guide surgical resection.
The advantage for using an NIR fluorescent agent such as ICG over a blue intravital dye is the opportunity to detect subsurface lymph nodes or tissues that may not otherwise be detected intraoperatively within the surgeon's FOV. While ICG fluorescence remains limited in tissue penetration, diagnostic nuclear imaging remains the hallmark for assessing tumour-draining deep lymph nodes in a pre-operative fashion.
Manual Lymphatic Drainage Course
As a result, in the majority of studies using ICG fluorescence navigation for localizing tumour draining lymph nodes, it is used intraoperatively, rather than non-invasively, in a pre-operative setting as reviewed by Vahrmeijer et al. It is important to note that because ICG is not cancer specific, it cannot be used for NIRF detection of cancer-positive lymph nodes, which is the goal of emerging NIRF molecular imaging agents. – A recent review of NIRF molecular imaging agents for identification of tumour margins during cancer surgery is not contained herein but rather in Vahrmeijer et al.
The following sections detail the performance of the various devices used intraoperatively for SLN detection. Sentinel lymph node mapping in breast cancerThe majority of studies using ICG fluorescence imaging for lymph node detection are performed in breast cancer as summarized in. Kitai et al first utilized the fluorescence properties of ICG for SLN detection in patients with early breast cancer using the PDE prototype device using 25 mg of ICG in 5 ml of water. Intraoperatively, sentinel nodes were found by visually following the green-stained lymphatic vessels to the first-draining SLNs that were fluorescent.
Since then, comparative studies have been performed to assess performance of ICG, blue dye and radioisotope alone or in combination using a range of devices and ICG doses. –,–,– In general, reports are mixed but show that fluorescence imaging provides higher detection rate and lower false-negative rate than do blue dye and radioisotope methods, and the combination of fluorescence imaging with blue dye visualization was found to be superior to the blue dye method alone., Moreover, the combination of fluorescence imaging with blue dye visualization had the highest sensitivity method, avoiding the need of the radioisotope method, and in another study, no benefit was found in using the blue dye when ICG was combined with radioisotope method. Investigators who used the mini-FLARE device often combined ICG with human serum albumin (HSA) to improve quantum yield and SLN retention of ICG fluorophore., Addition of HSA to ICG was not found to significantly alter SLN detection rate when the PDE device was used for detection. In another study of 134 patients with breast cancer, detection of SLNs from ICG was not found to be superior to the radiotracer method using the PDE device. StudyNumber of subjectsMean age of subjects (years)Total dose of ICGInjection siteDeviceOperative time (min)Averaged number of SLNsDetection rate (%)CommentsKitai et al1856.925 mg in 5 mlSubcutaneous periareolarPrototype of PDE10 µg)87 (dose 10 µg)Determine the minimum dose needed for visualization of lymph drainage pathwaysMurawa et al3056 (27–84)5 mg in 1 ml, 10 mg in 2 ml, 15 mg in 3 mlIntradermal periareolarIC-View5–101.7529/30 (97)Visualization of lymphatic vessels depends on the different doses of ICG. ICG is superior to radioisotopeTroyan et al661 (51–65)0.0124 mg in 1.6 mlPeritumourMini-FLARE™51.5 (1–4)9/10The use of ICG:HSA detected by mini-FLARESugie et al41157.9 (30–91)5 mg in 1 mlPeriareolarPDE–2.3 (1–9)408/411 (99)ICG is superior to blue dyeHirche et al4358.4 (27–83)11 mg in 2.2 mlSubareolarIC-View5–152.0 (1–3)42/43 (98)–Hojo et al14157.6 (34–83)10 mg in 2 mlIntradermal peritumour and subareolarPDE53.8140/141 (99)Comparison of ICG, patent blue and radioisotope.
Skin cancerStudies using ICG fluorescence imaging for SLN detection in skin cancer are summarized in. Fujiwara et al first reported the use of fluorescence method to identify the SLN successfully in melanoma and squamous cell carcinoma of the skin. Moreover, they found that use of fluorescence method avoids the “shine-through” phenomenon seen in lymphoscintigraphy, thereby providing high detection rate of the cervical SLN. In general, 0.5 mg in 0.1 ml dose of ICG was injected intradermally at various sites around the tumour, with a total dose volume ranging from 0.4 to 1.0 ml. To improve fluorescence signal, ICG:HSA was also used in melanoma SLN detection, and the ICG:HSA dose escalation clinical trial was also performed. In addition, the effectiveness and limitations of fluorescence detection of lymph nodes were evaluated with transcutaneous imaging, and imaging within the surgical FOV and of the excised tissues. Studies showed a lower detection of lymph nodes from transcutaneous as opposed to from within the surgical FOV or from resected tissues containing lymph nodes.
Manual Lymphatic Drainage Classes
It was observed that transcutaneous SLN detection rate could be improved by manual manipulation of overlying tissues or by increasing the dose of ICG. Other opportunities for improving detection could result from improving device sensitivity. StudyNumber of subjectsMean age of subjects (years)Total dose of ICGInjection siteDeviceOperative time (min)Averaged number of SLNsDetection rate (%)CommentsFujiwara et al1068 (34–80)3–5 mg in 0.6–1.0 mlIntradermal peritumourPDE52.624/25 (96)The dose escalation clinical trialNakamura et al1266 (32–85)3.0 mg in 0.6 mlIntradermal peritumour––3.295The improvement in the detection rate of cervical sentinel nodes in head and neck skin cancer using fluorescence method in combination with the standard technique. Other cancersStudies using ICG fluorescence imaging for SLN detection in other cancers are summarized in. Miyashiro et al used the fluorescence method to detect the SLN of gastric cancer in open gastrectomy, and ICG was injected endoscopically around the tumour 1 day before surgery. Later studies showed that pre-operative, endoscopic ICG injection provides a larger averaged number of SLNs, a higher accuracy rate and a lower false-negative rate than does intraoperative ICG injection in cT1-stage gastric cancer. Some investigators showed the possibility of SLN mapping guided by ICG fluorescence imaging during laparoscopy-assisted gastrectomy.
The adequate dose of ICG injected submucosally on the day before operation has been reported to be four doses of 0.5 ml containing 50 µg in 1 ml ICG for SLN mapping using HyperEye Medical System in the gastric cancer surgery. The NIRF method has also been applied for SLN mapping in rectal, anal, colon, and colorectal cancers with acceptable detection rates.
Moreover, the SLN could be detected not only in T1 disease but also in T2 and T3 disease. In these studies, the total dose of ICG ranged from 14 mg in 2.8 ml to 25 mg in 5 ml injected around the tumours. The da Vinci, integrated with FIREFLY, has been used for SNL mapping in bladder and prostate cancer., Recently, the application of the NIRF method for SLN detection was attempted in cervical cancer, showing a similar detection rate as that using the blue dye and radioisotope method. As in breast and skin cancers, 1.6 ml of ICG:HSA was injected around the tumour for SLN mapping in patients with cervical cancer.
Further studies demonstrated that there is no advantage of ICG:HSA over ICG alone for SLN detection in early stage cervical cancer, thus avoiding the added cost and complexity in using ICG:HSA. SLN mapping has also been demonstrated using robotically assisted endoscopic NIR imaging after cervical injection of appropriate dose of ICG around the tumour. The NIRF method for SLN mapping has been introduced in other types of cancers, such as vulvar and endometrial cancer, – head and neck cancer – and non-small-cell lung cancer, with high detection rate. LYMPHATIC IMAGINGClinical lymphatic imaging studies using NIRF imaging systems are summarized in.
NIRF imaging has been used to characterize lymphoedema of the upper and lower extremities using both PDE,–,–, and FDPM devices., Interpretation of NIRF images include aberrant lymphatic vasculature as seen comparatively to normal lymphatic vasculature in (Supplementary Video A), impaired transit time measurements ( i.e., the time between ICG injection and its appearance in the nearest draining lymph nodes) using the PDE device; as well as measurements of reflux or impaired lymphatic propulsion using the FDPM device (; Supplementary Video B). Using the PDE device, Mihara et al showed NIRF imaging to be a superior diagnostic to lymphoscintigraphy when evaluating for lymphoedema. NIRF imaging has also been used to detect lymphatic abnormalities before the onset of swelling symptoms using the FDPM imager, earlier than lymphoscintigraphy and following cancer surgery. Using both devices, multiple intradermal injections of single ICG doses were used for lymphatic mapping, typically 0.025 mg in 0.1 ml saline using the FDPM imager and 0.5 mg in 0.1 ml water using the PDE device.
Assessment of lymphatic architecture and transport was also performed with NIRF imaging devices in subjects with head and neck lymphoedema, and used in the treatment setting to assess the effect of lymphoedema treatments, i.e. Manual lymphatic drainage and pneumatic compression devices, on lymphatic function., The results suggest the ability to use NIRF imaging to direct therapy and to stratify which subjects could benefit by these non-surgical therapies on the basis of stimulated lymphatic pumping or lymphatic vessel recruitment. (a) Static near-infrared fluorescence (NIRF) imaging of indocyanine green (ICG)-laden lymphatics in the arm of a normal subject. Supplementary Video A shows increased lymphatic contractile transport in response to manual lymphatic drainage applied by a trained therapist. (b) Static NIRF imaging of ICG-laden lymphatics in the arm of a breast cancer survivor with lymphoedema in the right extremity as depicted in (c).
Supplementary Video B shows the comparative lack of lymphatic propulsion compared with that shown in the arm of a normal subject shown in (a). Reproduced from Tan et al, with permission from Elsevier. PHANTOMS FOR ASSESSING CLINICAL PERFORMANCECurrently, the success of NIRF imaging is based upon the ability of the device to detect ICG in humans, as outlined in –, with the expectation that “first-in-human” molecularly targeted imaging agents can likewise be detected by these devices already employed to detect ICG. ICG is comparatively dim compared with other emerging NIR fluorophores, such as IRDye800® (Li-Cor Biosciences, Lincoln, NE) and others, which promise brighter fluorescent signals and the ability to detect targeted tissues deeper and with smaller concentrations than is used for haemo- and lymphovascular imaging of ICG.
Device performance is critical and depends upon optimal excitation of NIRF imaging agents, rejection of backscattered excitation and ambient light, and selective collection of fluorescence emanating from the fluorophore. While instrument design differs widely , and NIRF molecularly targeted imaging agents can be expected to have broad spectral characteristics that make them amendable to different devices, there remains no systematic measurement procedure and test to enable prediction that a given molecular imaging agent can be detected in humans by a given NIRF imaging device. Furthermore, as technologies evolve and as NIRF imaging device components change, there remains no standardized means that could track device improvements over time and establish clinical performance without involving clinical trials that are often costly. There is also no standardized measurement and approach to qualify the consistent, equivalent operational status of a single device as a function of clinical usage time. Hence, there is an urgent need to develop working standards that are stable over time and enable qualification of imaging device performance and that decouple the device and drug regulatory processes. For example, if a “first-in-humans” imaging agent fails to demark a diseased tissue, how is one to determine whether it was the imaging agent that failed to target the diseased tissue or whether the imaging device was not sensitive enough for its detection? The ICG dose escalation studies by Sevick-Muraca et al and Mieog et al provide important information for setting the dosing and detection levels of imaging agents to begin safety and toxicity studies for eventual translation but inevitably are specific to the particular imaging device used.Many groups have embarked on the process to construct solid phantoms for quantifying the performance of complete NIRF imaging systems, as well as for comparing systems.
While past work has focused upon adding fluorophore or imaging agent in milk or intralipid solutions, these liquid standards have a finite shelf life, are not often replicable and require comprehensive characterization for each use. Summarizes the developmental efforts found in the literature that seek to establish solid phantoms in which a fluorophore or luminescent material is embedded in a solid medium that mimics or exaggerates tissue-scattering properties.,– These phantoms are essential to sensitively and accurately detect changes in device performance during manufacture, installation and operation in the clinic. Because ambient light can change with time in the environment, the use of the standards at the point of care can define the expected performance of the device. Hence, if the ICG is not detected during lymphatic mapping in a specific site, then one can safety assume that there are no functional lymphatics to be visualized. Likewise, if a molecular imaging agent is not detected, one can conclude that the molecular imaging agent was not present in or subsurface to the FOV.
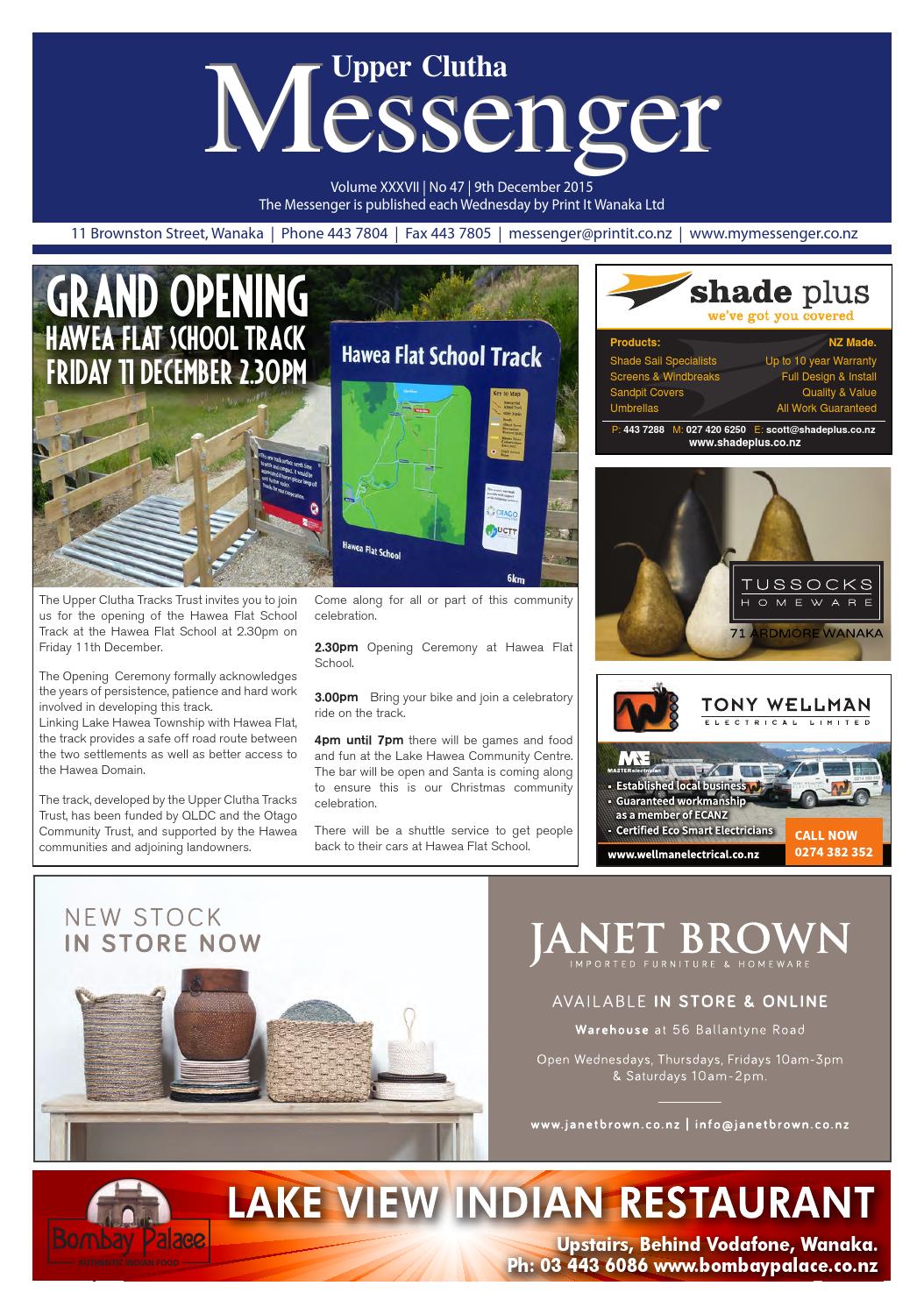
Long-term stability (1 year) of these phantoms remains elusive, and while promising candidates are under development, as shown in, there needs to be an adoption of an industry standard to promote further technological evolution of NIRF devices and their use with emerging NIRF imaging agents. SUMMARYNIRF imaging is rapidly emerging for clinical characterization of the lymphatic vasculature, whether for SLN mapping in cancer surgeries or for assessing lymphatic vessel architecture and function in health and a variety of diseases. Herein, we summarized the performance variety of NIRF imaging devices used in the clinic to detect ICG, the only NIR excitable dye currently used in humans.
The performance of these devices, while variable, offers promise for the emerging NIRF molecular imaging agents that promise profound impact in surgical and non-surgical applications. Now that there is a plethora of NIRF devices in the clinic and a range of ICG doses used as summarized herein, a critical evaluation of the efficient approaches to evolve to the next step of using “first-in-humans,” molecularly targeted imaging agents is needed.